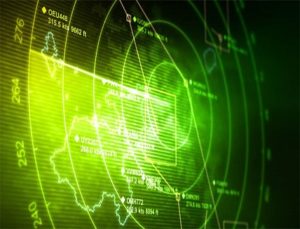
The aerospace and defense sector is witnessing major innovations on a global scale, with aspects such as electronic countermeasures, techniques for intelligence, surveillance and reconnaissance systems evolving at a rapid pace. In India, the move has been towards making the country self-reliant in defence production, as 70% of all defence requirements are currently met through imports. A positive sentiment towards innovation, however, exists in India, just as strongly as other countries.
Given this scenario, engineering tasks continue to be ever-evolving and more challenging. While system complexity may be increasing, timelines and budgets cannot adapt themselves at a similar pace. Yet, underlying technologies are stepping up their game with the ability to design more sophisticated systems more quickly. Four recent innovations will have huge enabling impacts on radar technology over the next several years:
1. Gallium Nitride for Front-End Components
Gallium Nitride (GaN), often considered one of the most important semiconductor innovations since silicon, is a material that is capable of operating at a much higher voltage than conventional semiconductor materials. Due to the resulting increase in efficiency, products such as RF power amplifiers and attenuators that use GaN consume less power and produce less heat. These advantages have led to a much larger influx of GaN-based RF component suppliers and production-ready, reliable products such as GaN-based amplifiers. This material has also aided in rejuvenating the Indian semiconductor market, with the government supporting its fabrication in centers such as the Indian Institute of Science (IISc) over the past 2 decades.
This technology is important for the evolution of active electronically scanned array (AESA) radar systems which consist of fully active arrays with hundreds or thousands of antennas, each with its own phase and gain control. These radar systems steer beams electronically using a phased array of transmitters and receivers without physically moving the antenna. The popularity of these radar systems centers directly on their increased power on target, spatial resolution and improved robustness compared with other conventional radars. For example, if one element in the array fails, the radar continues to operate. The increased use of GaN amplifiers in AESA radars should offer better performance, achieving equivalent output power with smaller form factors and requiring less cooling.
Figure 1. AESA Radar Architecture
As applications and solutions using GaN technology grow more advanced, component-level test results at a system level become more critical. Traditional methods of component test using vector network analyzers provide an accurate and narrow band view of forward and reflected gain and phase. However, the continuous wave (CW) stimulus in this popular method does not accurately reflect the actual signal environment the component is ultimately used in. As an alternative, the wide band flexibility of vector signal analyzers and vector signal generators can be used to create pulses and modulated stimuli more representative of real-world applications and their environments. Combining this capability with S-parameter analysis is an increasingly more strategic method of testing at a component level.
2. High-Speed Data Converters for Transmit and Receive
Another major advancement has been in converter technology where the latest analog-to-digital converters (ADCs) and digital-to-analog converters (DACs) being released exceed the speed of their predecessors by a large degree. Such a scenario holds great promise for radar as the wider bandwidth not only allows for better spatial resolution but also makes it possible to implement innovative techniques such as moving between frequencies to avoid detection or perform a dual role as a communications system and a radar simultaneously.
These converters are even fast enough to perform “direct RF sampling,” which means sampling with enough speed to acquire RF signals directly without up- or down-converting. This ability is of importance to AESA radars, as it allows for the reduction of the size and cost of antennae by eliminating mixers and local oscillators.
Figure 2. Heterodyne Versus Direct RF Sampling Architecture
3. Evolving FPGA Technology for Cognitive Techniques
FPGA technology is another element that continues to improve annually, with the computational capability of today’s FPGAs being a distinct improvement over its predecessors of five years. Engineers today apply machine learning techniques to render radars more responsive to their environment. Through this, radars can now perform new techniques like automatically recognizing different targets, or adjusting their operating frequency or waveform based on their surroundings.

Figure 3. Machine Learning Techniques Implemented on the FPGA Within Cognitive Radar
In addition, higher level FPGA programming tools are becoming more capable, making it easier to port algorithms to FPGAs, which is a great advantage for engineers and scientists who do not have previous hardware description language (HDL) expertise or who have tight timelines.
4. High-Bandwidth Data Buses for Sensor Fusion
Another key technology that’s paved the way for radar innovation is the evolution towards higher bandwidth data buses such as PCI Express Gen3 and Xilinx Aurora. Using these buses allows for data aggregations from multiple sensors for centralized processing.
Similar to sensor fusion in autonomous vehicles, fighter jets such as the F-35 too can combine data from radars, electronic countermeasure devices, communications devices and other sensors to provide better situational awareness to pilots.
Figure 4. Aggregating Sensor Data From Multiple ISR Sensors for Centralized Processing Using High-Speed Data Buses With the rapid evolution of these underlying technologies, it is mandatory that radar techniques and architectures too evolve concurrently. With technology continuing to advance, systems must evolve to keep pace. With the availability of both design talent and an intimate knowledge in-house, developing fully custom hardware and software for radar prototypes and test systems internally is a viable option to achieve the required customization and performance. They must, however, overcome challenges such as long-term maintenance responsibility and opportunity cost before they are truly applicable.

As FPGAs and rapid adoption of new converters and streaming technologies in modular form factors becomes more common, COTS solutions can now both meet specification requirements as well as offer flexibility to ensure systems achieve the longevity required for longer life cycles. By rapidly incorporating these technologies in modular, COTS form factors, companies today enable engineers to meet the evolving requirements of advanced radar systems while also meeting strict timelines and budgets.